Using aluminum and copper for battery tab to cell welding improves electrical efficiency and battery module/battery pack performance.
New Laser Sources Improve Battery Performance by Enabling Dissimilar Metal Joining
David Van de Wall, Mark Boyle | AMADA MIYACHI
As the world becomes more mobile and components become smaller, the demand for longer lasting batteries has been increasing. Increasing battery life requires lower electrical resistance to reduce loss. New laser sources, including single mode fiber lasers and lasers with nanosecond pulses, provide an important opportunity to reduce resistance by enabling the joining of aluminum and copper battery tab material – reducing resistance and improving performance. Tests have shown the technology works well with metal thickness of less than 0.25 millimeters (mm), providing good pull and peel strengths in these dissimilar joints.
A brief history of battery pack manufacturing
This article concentrates on commonly used cylindrical cell batteries to explain the concept of dissimilar metal joining with lasers. The same technology can also be used for some other battery types, including pouch cells, which typically feature one aluminum and one copper tab that requires an electrical connection to the busbars.
Most cylindrical battery cans are made of cold rolled steel (CRS) that has been nickel-plated for corrosion resistance. Figure 1 shows one of the most common cells – lithium ion 18650. To connect multiple cells together, or connect one cell to outside equipment, a strap or tab must be connected to the battery.
Figure 1- Typical Cylindrical 18650 Battery with its terminals
Historically, tabs were made of nickel, stainless steel, nickel plated steel, or Hilumin® (an electro nickel-plated diffusion annealed steel strip) due to their similar properties and the ease with which they can create a fusion bond. These materials join well in a variety of welding techniques, including resistance spot welding, pulsed arc welding, and laser welding.
As the demand for battery and battery pack performance increases, new materials are being considered to improve current delivery. When we assume a certain type of battery is being used, the material of the two terminals of the battery is a given and can’t be changed by the battery module manufacturer. So a further optimizing of the electrical performance of the battery module requires consideration of dissimilar metal bonding to allow the usage of highly electrical conductive battery tabs to the battery terminals.
The benefits of dissimilar joining for battery applications
Designers of battery packs for hybrid and electric automobiles, motorcycles, buses, heavy industrial vehicles, and hand-held power tools are looking for more energy to support the vehicle or device and increase its life. To do this, they must change the batteries’ electrode, isolator, and electrolyte, and lower its internal resistance to reduce energy losses on a battery cell level. The lower the loss, the more efficiently energy is stored in the battery. These improved batteries store more energy and can also charge and discharge faster, as measured by the battery’s “C” rate– the ratio between the charge/discharge acceptance and its capacity.
The higher voltages and currents generated by improved batteries means the battery interconnection (battery tab) must improve its electrical conductivity. The higher electrical conductivity results in lower electrical losses when charging and discharging, so more electrical energy is available for the vehicle or device powered by the pack. By improving the electrical conductivity, the pack also stays cooler during operation, which provides additional performance and battery lifetime benefits. Last, but not least, improving battery performance lowers costs, because fewer batteries are required for a particular performance level.
The three options for improving battery interconnect performance are using thicker tabs to carry more current, using different tab materials (for example, copper or aluminum) with higher conductivity, or creating a larger tab to battery pole contacting area.
In the past, steel and nickel-based materials were commonly welded to the CRS terminals. However, more recently, as batteries have move towards higher capacity and higher “C” values, designers began looking at using aluminum or copper materials for tabs to reduce electrical losses. See Figure 2.
Challenges of using traditional Nd:YAG lasers for welding dissimilar metals
This rise in the use of dissimilar material welding comes with a variety of challenges for traditional welding options like pulsed Nd:YAG (neodymium-doped yttrium aluminum garnet) lasers, which typically make spots that are 0.5 mm in diameter with one pulse. Each weld pulse takes between 1 and 10 milliseconds; depending on its power level, the laser can generate about 10 of these pulses every second. To create a joint with sufficient electrical and mechanical properties, between 2 and 50 welding spots must be placed on each battery pole. See Figure 3.
This method worked well for the traditional battery tab materials because of their weldability. However, welding of dissimilar materials is significantly more challenging because of:
-
Different melting temperatures (see Table 1)
-
Different thermal expansion coefficients
-
Different absorption coefficient of laser light
-
Incompatible chemistry and atomic structures
Joining aluminum to stainless steel has always been an impossible welding combination. The mixing of these two metals creates a brittle intermetallic zone leading to weld cracks.
Innovative laser welding technologies
New laser technologies are rewriting the textbooks on which materials can be joined together. Single mode fiber lasers and lasers with nanosecond pulses are joining new combinations of metal that were previously not considered compatible.
The single mode laser can be focused to spot diameters of 20-50 microns – smaller than the diameter of a human hair! This makes a very small welding line of 20-50 microns wide, which can be pulsed like “a hot knife through butter” to make a weld. The linear speed of this laser beam movement is typically in the 100 to 1000 mm/sec (4-inch-40-inch/second) range. See Figure 4.
To create sufficient joint area, the length of this joint needs to be 10 to 100 mm per battery pole. To fit this onto the 3 to 6 mm diameter available on a battery pole, the line must be in a spiral shape or concentric circles, rather than spots. Using a galvo scanning weld head, the total weld can be very fast; it takes about 50 milliseconds of weld time.
Another method of welding dissimilar metals is to concentrate the laser energy in time by using nanosecond pulsed fiber welding instead of the traditional millisecond pulse. The weld pulses are typically 100,000 times shorter, providing energy in a much shorter period. This increases the laser peak power level by the same amount. The spot size is also very small, about 30 to 40 microns. The high peak power on a small area results in such a high peak power density that all metals are molten. This process can be best described as “pushing a hot needle in the material and pulling it out again.” The spots are very small, so a great many spots are needed, typically 10,000 or more. As the timeframe for creating one spot is very small, the laser can be fired at high repetition rates, typically 30 kilohertz (kHz) and above.
The best method of getting enough weld spots with the nanosecond laser on a small area like a battery pole is to make a line coiled into a spiral. This spiral is typically welded from the inside to the outside, so the laser beam always “sees” a fresh and cold piece of metal in front of it. If the laser went from the outside to the inside, there would be heat build-up and increased penetration depth towards the center, as the part heats up during the welding.
Figure 5 shows an example of a spiral weld made on aluminum tab material. Testing showed single layer shear strengths of around 44 N (10 pounds) and double layer shear strength at around 88 N (20 lbs.).
Figure 6 is an application photo showing an aluminum battery tab welded to a nickel-plated cold rolled steel can using the spiral weld technique. A closer look at the cross sections reveals that the spot welds do not show the characteristic form of conventional pulsed spot welds – they more closely resemble multi‐staking. The intermetallic zone was less than 10 microns. Pull strength was good and peel strength was adequate. There was barbed solidification of aluminum into steel.
One example of the new technology is AMADA MIYACHI’s 70-Watt LMF70-HP OEM fiber laser, which can be integrated into the LMWS pulsed fiber laser welding system. The new technology is an advanced processing system for welding battery cans with wall thicknesses of 300-350 microns. It features shorter pulses, resulting in less mixing of materials, and hence less of an intermetallic zone and less brittleness with aluminum tab materials. This laser solution is good for contract manufacturing settings, where operators may be producing one product one day and something different the next. The XY galvo program quickly positions welds; a new program can be loaded in as little as five minutes, and operators can be ready to start the next product.
New battery tab welding technologies widen the design portfolio by providing good solutions for dissimilar material joining
The new nanosecond pulsed fiber laser and the single mode fiber laser provide opportunities for dissimilar metal joining and show great potential as a solution for welding battery tabs to cells. Allowing for aluminum and copper tab material, which reduce electrical resistance and improve battery performance, the new technology shows great promise for a wide range of industries.
The content & opinions in this article are the author’s and do not necessarily represent the views of ManufacturingTomorrow
Comments (0)
This post does not have any comments. Be the first to leave a comment below.
Featured Product
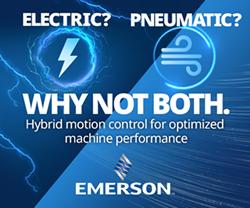