Driven by the demand for smaller and smaller consumer products and semiconductor devices, manufacturers require thin planar optics for an array of applications.
Overcoming Pitfalls When Measuring Thin, Parallel Optics
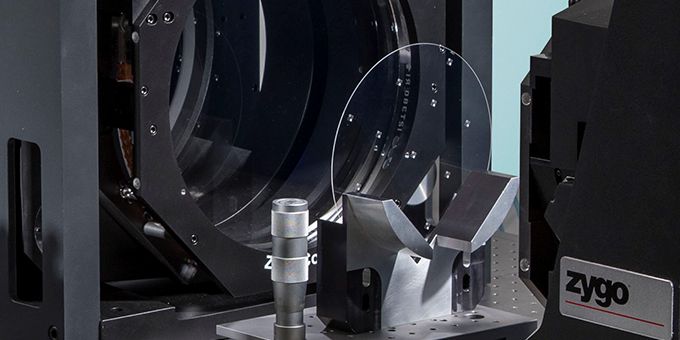
Dan Musinski | Zygo Corporation
Driven by the demand for smaller and smaller consumer products and semiconductor devices, manufacturers require thin planar optics for an array of applications. This puts the responsibility on the material and optics manufacturers to ensure the glass is flat and free of material deformities which can cause distortion and affect end-use functionality.
This places a huge burden on metrology tools that need to measure and confirm the uniformity of thin planar optics thereby proving that they are fit for purpose.
Inherently, the measurement of thin parallel optical surfaces can be extremely taxing. Such optics are characterized by the fact that they are less than a few millimeters thick, and this means that the front and back surfaces are very close together. Because of this, standard mechanical phase shifting interferometry (PSI) finds it difficult to discern between the surfaces.
A more advanced solution is Fourier-transform phase-shifting interferometry (FTPSI), which enables easy characterization of the front and back surface, optical thickness variation, and material homogeneity of thin plane parallel glass. FTPSI makes it possible to distinguish between the front and back surfaces and characterize the quality of both in a single measurement, even if they are less than a millimeter thick.
WHY FTPSI?
To understand why FTPSI is the preferred technique for measuring thin parallel optics, we need to take a closer look at the alternative traditional measurement techniques and see where they fall short.
If we consider PSI which works by passing a light beam through an ideal reference optic (called a transmission flat [TF]), to the part under test, we see that this technique cannot distinguish between the front and back surfaces of a thin parallel optic.
When properly aligned, the TF and the part under test create an interference pattern, recorded as an interferogram. The metrology software analyzes the height variations produced by the phase shifts and reconstructs the surface wavefront, which represents the difference in height between the TF and the test part.
When the front surface of a thin, parallel part is aligned, a second reflection is typically returned to the interferometer from the back surface. This results in a complex fringe pattern created by multiple, overlapping interferograms that cannot be accurately analyzed using PSI. (See Figure 1.)
Complex fringe pattern caused by multiple surface reflections
There are actions that can be taken to improve the situation, but they are time consuming and add unnecessary and potentially damaging steps into the overall process. These include applying black paint to the back surface to extinguish its reflection, coloring with a dark colored marker, or spreading petroleum jelly on the surface.
The FTPSI method negates the necessity to manually manipulate the back surface of the thin optic to undertake meaningful and accurate measurements. Instead, FTPSI uses the refection from the back surface to gain more information about the thin optical component in a single measurement.
This is possible because FTPSI does not require mechanical motion within the test cavity to create the interferograms. Instead, FTPSI relies on modulation of the wavelength of the laser source to enable the measurement. Each cavity in the optical path in an FTPSI acquisition produces a unique interference frequency that defines its cavity length, and this enables a clear delineation and accurate characterization of the surface. Algorithms can then analyze both surfaces and characterize their form independently. (See Figure 2)
Front (2a) and back (2b) surface form results of a 1.2 mm thick sample shown in figure one.
3- and 4-SURFACE FTPSI
Let’s start with the basics. A TF — as mentioned above — is used with an interferometer to establish a plano reference for a surface or transmitted wavefront measurement. A reference flat (RF) is a high-quality optical surface that is used to direct a measurement beam with minimal effect on the overall wavefront.
The simplest FTPSI measurement is a three-surface configuration that consists of the TF — surface 1 ‚ and the test part — surfaces 2 and 3 (See Figure 3). In this configuration, a back-surface result is provided but it includes material non-uniformities due to the measurement beam passing through the material of the test part.
Example of a 3-surface and 4-surface configuration
For higher accuracy measurements of the back surface, a four-surface configuration can be used by placing a RF — surface 4 — behind the test optic. In this configuration, the form of surface 3 is compared to the known RF. This configuration creates a second test cavity between the back surface and the RF and provides a direct measurement of the back surface without the uncertainty of the material in the part.
A single FTPSI measurement with both the three- and the four-surface cavity configurations includes a thickness deviation result, which is a full-surface map of the material thickness across the test part.
MATERIAL HOMOGENEITY
The four-surface cavity configuration described above enables the characterization of the material homogeneity of the test part, a unique feature of the FTPSI technology.
The homogeneity information can be obtained by first measuring the cavity with the test part inside, then removing the part from the cavity and performing an “empty cavity” measurement, allowing a comparison between the TF and the RF. Unlike other homogeneity measurement techniques that only provide the nonlinear component, an FTPSI result maintains a fixed cavity and, therefore, can provide both the nonlinear and linear components of the material homogeneity. The linear portion is critical for applications that are sensitive to beam pointing, as the result can be used to predict how a beam deviates when passing through the test part.
ACCURACY
As with all interferometric test methods the measurement uncertainty is based on a number of factors including the quality of the reference optics, stability of the measurement environment and mounting techniques.
For parts less than 6 inches in diameter (150mm) the reference optic peak-to-valley surface form can be of the order of 2.5% of the wavelength of the light used to make the measurement — λ/40. If the system, for example, has a laser emitting red light at a wavelength of 633 nm this corresponds to approximately 16 nm. In most cases this enables the resultant measurement to be well within the tolerance bandwidth for thin glass applications.
How the part is held in the test cavity is probably the most critical factor when measuring thin optics, more specifically the mounting technique and the mounting orientation. Simply clamping a thin optic can induce unwanted stress and cause the optic to bend. Differences in orientation can yield very different measurement results, especially for thin parts, due to gravity affects. Ideally, the part should be mounted in the same configuration in which it will be used in its end-use application to avoid unexpected differences between the designed intent and actual performance. (See Figure 4.)
Mounting examples for thin glass optics. The configuration in 4a is prone to be overtightened which can lead to distortion of the part, while the “V-block” image in 4b provides a low stress mounting solution.
SUMMARY
FTPSI is a compelling choice for optics manufacturers who need to ensure the quality of thin, parallel optics. Unlike conventional mechanical PSI, FTPSI can distinguish the front and back surfaces and characterize their corresponding surface information in a single, repeatable measurement. Thanks to advances in both equipment and algorithms FTPSI can characterize surface form, thickness deviation, and material homogeneity of optics that are less than 1 mm thick. Faced with the growing demand for thin, parallel optics, and the challenges involved in accurately measuring those optics, FTPSI overcomes the limitations of previous methods. Its strength in characterization along with its ease-of-use makes it a good choice for optical metrology.
Zygo Corporation is owned by AMETEK, Inc., a leading global manufacturer of electronic instruments and electromechanical devices with annual sales of approximately $5 billion. ZYGO is a worldwide supplier of optical metrology instruments, high-precision optical components, and complex electro-optical systems, and Its products employ various optical phase and analysis techniques for measuring displacement, surface shape and texture, and film thickness.
The content & opinions in this article are the author’s and do not necessarily represent the views of ManufacturingTomorrow
Comments (0)
This post does not have any comments. Be the first to leave a comment below.
Featured Product
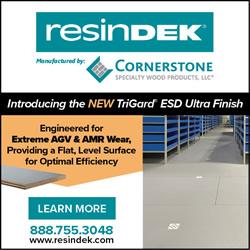